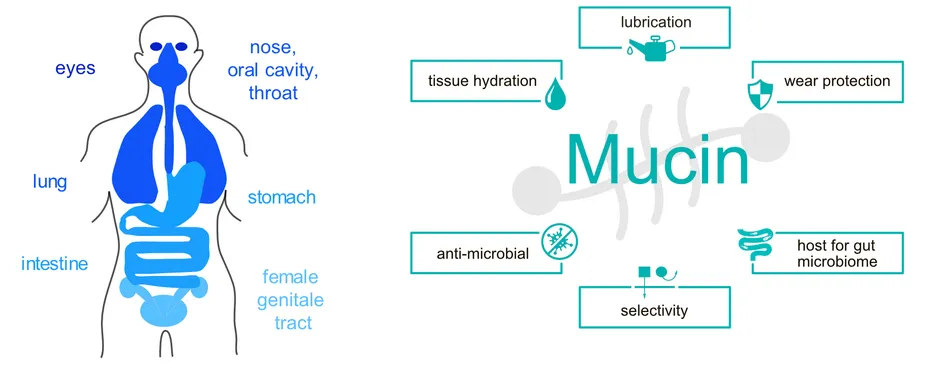
As part of their physiological function, many epithelial tissues in the human body (Figure 1) are exposed to tribological shear stress. Examples include blinking, swallowing of food, and copulation. To prevent mechanical damage of the involved tissues during those processes and to guarantee an unimpeded functionality of the related body functions, proper lubrication and wear protection is needed. This is accomplished by several variants of mucus - a viscoelastic hydrogel that covers all mucosal membranes of mammalians. [1] In addition to acting as lubricants, these mucus systems are also responsible for regulating the passage of molecules and particles towards the tissue in the gastrointestinal tract, protecting wet epithelial surfaces from dehydration, and serving as a defense layer against mucosal pathogens. [2,3] The key constituent of such mucus hydrogels are mucins, a class of high molecular weight (~2- 3 MDa) glycoproteins, which exhibit a highly complex molecular architecture. During the past years, we and others contributed to elucidating the structure-function relationship of mucin glycoproteins and evaluating the potential of using purified mucins in biomedical applications.
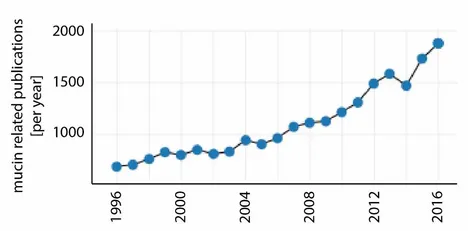
For the last 20 years, the number of mucin-related publications has been growing continuously. In 2016 alone, there were ~1800 articles published on this topic (Figure 2). On the one hand, this increasing interest in mucin might have been triggered by the realization that mucins play a key role in many physiological processes; moreover, alterations in the composition or function of mucosal systems often lead to illnesses including dry eye/dry mouth, cystic fibrosis or some forms of cancer. On the other hand, mucins have been shown to be very promising candidates for applications in the field of biomedical engineering [4].
Unfortunately, mucin research is still limited by the availability of functional mucins. Although the poor quality of commercial mucins is well-documented [5,6], many research groups still use commercial mucins in their studies. As we demonstrated recently [7], harsh conditions during industrial purification processes damage the mucin glycoproteins leaving corrupted molecules that have lost their unglycosylated termini – and those corrupted mucins have altered physico-chemical properties. Thus, results obtained with such damaged mucins are questionable, at best.
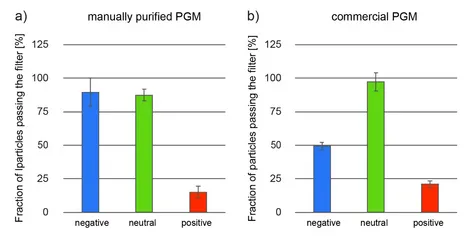
Selective properties of mucins:
One key function of the native mucus layer is to protect the underlying epithelial tissue from pathogens while allowing nutrients to pass [1]. Therefore, mucus has to possess selective properties, so it can distinguish benign food particles from harmful bacteria and viruses. To a large extent, this selective permeability can be established by mucins [8], e.g. via engaging in binding interactions with charged particles and molecules [1,9]. Indeed, cell culture experiments showed that in-lab purified gastric mucins possess strong anti-viral properties [10], and mucin gels have similar permeability properties as native mucus [11]. In the central part of the mucin glycoprotein, the molecule comprises a densely glycosylated region, and several carbohydrates found in this region (such as sialic acid or sulfated sugar residues) possess a strongly anionic character. Consistently, in-lab purified porcine gastric mucins (PGMs) have been shown to bind cationic particles while letting anionic and neutral particles pass (Figure 3a). However, commercial PGMs also show a strong affinity towards negatively charged particles (Figure 3b). [7, 12] This result agrees with our experimental finding, that the glycosylation pattern of these commercial mucins is incomplete: a partially exposed protein backbone of the mucin glycoprotein (this backbone carries many amino acids, which can be positively charged at neutral pH, i.e., lysine, histidine, and arginine) could explain the observed behavior.
.
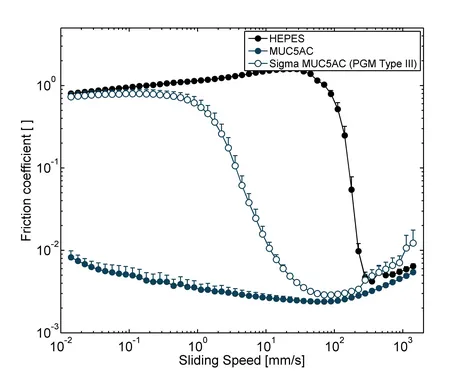
Lubricating properties of mucins:
In addition to their function as a component of selective barriers, mucins also lubricate epithelial surfaces and protect them from mechanical damage. Without proper lubrication, many physiological processes including chewing and swallowing of food, blinking or sexual intercourse would not be possible. The lubricity of mucin solutions originates from their ability to bind many water molecules [12] and to adsorb to various surfaces [1]. Already small amounts, typically ~0.1 % (w/v), of manually purified mucins can reduce friction in the physiologically most relevant boundary lubrication regime, i.e. at small sliding velocities, by up to two orders of magnitude compared to buffer alone [13]. However, commercial PGMs are vastly inferior lubricants and merely shift the transition from the boundary lubrication to mixed lubrication without reducing boundary friction (Figure 4). Also the lubricity of commercial salivary mucins is inferior that that of in-lab purified salivary mucins [14]. Together, this shows the importance of using highly functional mucins for studying biotribological effects.
Conclusion:
In the near future, there will be an increasing need for functional mucins both for research and for developing medical products. However, commercially available mucins (especially porcine gastric mucins) do not offer the required quality for either purpose. At this point, it seems that highly functional mucins can only be obtained by careful in-lab purification. Yet, the manual purification process of mucins can be time consuming and thus cost-intensive. Based on our experience with the purification and characterization of mucin glycoproteins, we have recently developed at set of standardized control tests that allows us to produce highly functional mucins a constantly high level of quality [16]. Moreover, we keep optimizing and scaling-up the purification process of porcine gastric mucins.
References:
[1] Bansil, R. & Turner, B. S. Mucin structure, aggregation, physiological functions and biomedical applications. Current Opinion in Colloid & Interface Science 11, 164-170 (2006).
[2] Linden, S. K., Sutton, P., Karlsson, N. G., Korolik, V. & McGuckin, M. A. Mucins in the mucosal barrier to infection. Mucosal Immunol 1, 183-197 (2008).
[3] McGuckin, M. A., Linden, S. K., Sutton, P. & Florin, T. H. Mucin dynamics and enteric pathogens. Nat Rev Microbiol 9, 265-278, doi:10.1038/nrmicro2538 (2011).
[4] Petrou, G. & Crouzier, T. Mucins as multifunctional building blocks of biomaterials. Biomaterials Science, doi:10.1039/C8BM00471D (2018).
[5] Schömig, V. J. et al. An optimized purification process for porcine gastric mucin with preservation of its native functional properties. RSC Advances 6, 44932-44943 (2016).
[6] Kocevar-Nared, J., Kristl, J., Smid-Korbar, J. Comparative rheological investigation of crude gastric mucin and natural gastric mucus. Biomaterials 18, 677-681 (1997).
[7] M. Marczynski, K. Jiang, M. Blakeley, V. Srivastava, F. Vilaplana, T. Crouzier, and O. Lieleg, Structural alterations of mucins are associated with losses in functionality. Biomacromolecules, 22 (4), 1600-1613 (2021)
[8] Witten, J. & Ribbeck, K. The particle in the spider's web: transport through biological hydrogels. Nanoscale 9, 8080-8095, doi:10.1039/C6NR09736G (2017).
[9] Lieleg, O. & Ribbeck, K. Biological hydrogels as selective diffusion barriers. Trends in Cell Biology 21, 543-551, doi:DOI 10.1016/j.tcb.2011.06.002 (2011).
[10] Lieleg, O., Lieleg, C., Bloom, J., Buck, C. B. & Ribbeck, K. Mucin biopolymers as broad-spectrum antiviral agents. Biomacromolecules 13, 1724-1732 (2012).
[11] Lieleg, O., Vladescu, I. & Ribbeck, K. Characterization of particle translocation through mucin hydrogels. Biophys J 98, 1782-1789, doi:10.1016/j.bpj.2010.01.012 (2010).
[12] Winkeljann, B., Käsdorf, B. T., Boekhoven, J. & Lieleg, O. Macromolecular Coating Enables Tunable Selectivity in a Porous PDMS Matrix. Macromolecular Bioscience 18, 1700311, doi:doi:10.1002/mabi.201700311 (2018).
[13] Ma, L., Gaisinskaya-Kipnis, A., Kampf, N. & Klein, J. Origins of hydration lubrication. Nature Communications 6, 6060 (2015).
[14] Käsdorf, B. T. et al. Mucin-Inspired Lubrication on Hydrophobic Surfaces. Biomacromolecules 18, 2454-2462 (2017).
[15] Biegler, M., Delius, J., Käsdorf, B. T., Hofmann, T. & Lieleg, O. Cationic astringents alter the tribological and rheological properties of human saliva and salivary mucin solutions. Biotribology 6, 12-20 (2016).
[16] M. Marczynski, C.A. Rickert, T. Fuhrmann, and O. Lieleg, An improved, filtration-based process to purify functional mucins from mucosal tissues with high yields. Separation and Purification Technology. DOI: 10.1016/j.seppur.2022.121209 (2022)