High-Performance Simulations of Wave Propagation for Structure Analysis of Concrete
The subproject BU focuses on the optimization of the Coda-Wave-Interferometry (CWI) technique for evaluating reinforced concrete components. It includes simulations at the specimen and component scale as well as the application of geophysical inversion techniques to investigate the influence of loads on wave propagation and determine the optimal placement of ultrasonic sensors. The goal is a qualitative and quantitative long-term evaluation of reinforced concrete components.
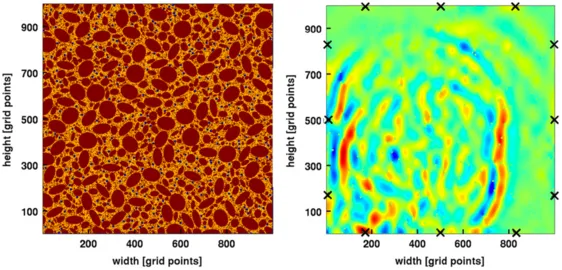
Left: Synthetic model (0.1 m x 0.1 m; 1000x1000 grid-points) of concrete which is similar to an X-ray CT of concrete. The cement paste (light brown) contains ellipsoidal aggregate particles (dark brown) and air voids (blue). Right: A snapshot of the vertical
displacement field originating from two ultrasound sources located at (400,300) and (400,500). The positions of the sensors are marked with black crosses.
Project description
It is experimentally demonstrated that ultrasonic waves are sensitive to different loadings of Reinforced Concrete (RC). A tool for the corresponding analysis is the Coda wave Interferometry (CWI). However, concrete is a strongly heterogeneous and densely packed composite material. Due to the high density of scattering constituents and inclusions, ultrasonic wave propagation in this material consists of a complex mixture of multiple scattering, mode conversion and diffusive energy transport. For a better understanding of the effect of aggregates, porosity, and of crack distribution on elastic wave propagation in concrete and to optimize inverse techniques it is useful to simulate the wave propagation and scattering process explicitly in the time domain. For this purpose, we use the Rotated Staggered Grid (RSG) finite-difference technique for solving the wave equations for elastic, anisotropic, and/or viscoelastic media.
The subproject BU of the research unit is subdivided into five Work Packages (WP).
The first one concentrates on ultrasound simulations as a service for collaborating partners (especially with RUB1, BAM and TUM2). With this task we continue the close collaboration on this issue during the first phase of the research unit. In the second work package we will further develop digital twins by X-Ray Computed Tomographic (XRCT) images. With this tool we estimate the influence of different loads (moisture, temperature, and mechanical load) to the microstructure of concrete. Image segmentation based on material knowledge is a crucial step in this procedure. By the transformation of several Digital Rock Physics approaches from geophysics to concrete we can estimate the corresponding influence on effective properties of concrete. In work package four we use the results of WP 3 to estimate the corresponding influence on the Coda signal. With the help of an international partner, we also will apply further machine learning techniques to interpret the coda signal in synthetic as well as in real structures. The fourth work package aims to extend the Time Reverse Imaging (TRI) technique for localization and characterization of Acoustic Events to an Active-TRI which will use the Coda signals to localize and characterize damage zones. The goal of WP 5 is to apply the machine learning techniques to identify the different loads (WP 3) and the Active-TRI technique to locate damage zones (WP4) to the test sides suggested in the main proposal of the research unit (Gänstorbrücke and a subway station in Munich). In summary we will optimize CWI with the help of high-performance computer simulations and the application of geophysical inversion techniques. Qualitative and quantitative long-term assessment of RC will be further developed.
Previous Results
In the first funding period, the CWI technique was used in sub-project BU to detect microcracks in concrete structures at an early stage. Numerical simulations of realistic concrete models were used to detect changes in ultrasound wave propagation in different damage scenarios.
Realistic concrete models with different grain size ranges were created and simulated using discrete elements to generate virtual mesostructural concrete models with microcracks. CWI was used to detect changes in ultrasound wave propagation in these models and differentiate between diffuse and localized damage. The results showed that CWI is a promising method for early detection of distributed microcracks in concrete structures, especially when long recording lengths and multiple optimally placed transducers are used.
With machine learning methods and a reflected coda-wave technique, a damaged zone could also be detected with a single sensor. For this purpose, a numerical model was created to investigate the effects of various factors such as scattering, noise, intrinsic damping, and sensor width on accuracy. The method can be applied as a supplement to traditional CWI and offers the possibility to use more advanced imaging techniques in this context.
Another result is the examined prediction of crack sizes in material samples using machine learning methods. Numerical models with different crack sizes were created and investigated using simulations of wave propagation. By evaluating the reflected and transmitted signals using artificial neural networks, a prediction accuracy of over 96% could be achieved. Certain signal parameters have proven to be particularly relevant for good predictions. The examined methods represent a promising tool for non-destructive testing of materials.